Space medicine is an area in aerospace medicine that focuses on the medical care of astronauts and spaceflight participants. The spaceflight environment poses many unique stressors to the human body, including G forces, microgravity, unusual atmospheres such as low pressure or high carbon dioxide, and space radiation. Space medicine applies space physiology, preventive medicine, primary care, emergency medicine, acute care medicine, austere medicine, public health, and toxicology to prevent and treat medical problems in space. This expertise is additionally used to inform vehicle systems design to minimize the risk to human health and performance while meeting mission objectives.
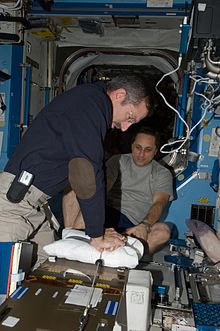
Astronautical hygiene is the application of science and technology to the prevention or control of exposure to the hazards that may cause astronaut ill health. Both these sciences work together to ensure that astronauts work in a safe environment. Medical consequences such as possible visual impairment and bone loss have been associated with human spaceflight.[2][3]
In October 2015, the NASA Office of Inspector General issued a health hazards report related to space exploration, including a human mission to Mars.[4][5]
History edit
Hubertus Strughold (1898–1987), a former Nazi physician and physiologist, was brought to the United States after World War II as part of Operation Paperclip.[6] He first coined the term "space medicine" in 1948 and was the first and only Professor of Space Medicine at the School of Aviation Medicine (SAM) at Randolph Air Force Base, Texas. In 1949, Strughold was made director of the Department of Space Medicine at the SAM (which is now the US Air Force School of Aerospace Medicine (USAFSAM) at Wright-Patterson Air Force Base, Ohio. He played an important role in developing the pressure suit worn by early American astronauts. He was a co-founder of the Space Medicine Branch of the Aerospace Medical Association in 1950. The aeromedical library at Brooks AFB was named after him in 1977, but later renamed because documents from the Nuremberg War Crimes Tribunal linked Strughold to medical experiments in which inmates of the Dachau concentration camp were tortured and killed.[7]
Soviet research into Space Medicine was centered at the Scientific Research Testing Institute of Aviation Medicine (NIIAM). In 1949, A.M. Vasilevsky, the Minister of Defense of the USSR, gave instructions via the initiative of Sergei Korolev to NIIAM to conduct biological and medical research. In 1951, NIIAM began to work on the first research work entitled "Physiological and hygienic substantiation of flight capabilities in special conditions", which formulated the main research tasks, the necessary requirements for pressurized cabins, life support systems, rescue and control and recording equipment. At the Korolev design bureau, they created rockets for lifting animals within 200–250 km and 500–600 km, and then began to talk about developing artificial satellites and launching a man into space.[8] Then in 1963 the Institute for Biomedical Problems (IMBP) was founded to undertake the study of space medicine.[9]
Animal testing edit
Before sending humans, space agencies used animals to study the effects of space travel on the body.[10] After several years of failed animal recoveries, an Aerobee rocket launch in September 1951 was the first safe return of a monkey and a group of mice from near space altitudes.[11] On 3 November 1957, Sputnik 2 became the first mission to carry a living animal to space, a dog named Laika. This flight and others suggested the possibility of safely flying in space within a controlled environment, and provided data on how living beings react to space flight.[10] Later flights with cameras to observe the animal subjects would show in flight conditions such as high-G and zero-G.[11] Russian tests yielded more valuable physiological data from the animal tests.[11]
On January 31, 1961, a chimpanzee named Ham was launched into a sub-orbital flight aboard a Mercury-Redstone Launch Vehicle. The flight was meant to model the planned mission of astronaut Alan Shepard. The mission planned to reach an altitude of 115 miles, and speeds up to 4400 miles per hour.[12] However, the actual flight reached 157 miles and a maximum speed of 5857 miles per hour.[12] During flight, Ham experienced 6.6 minutes of weightlessness. After splashing down in the Atlantic Ocean, Ham was recovered by the USS Donner.[13] He suffered only limited injuries during flight, only receiving a bruised nose.[14] Ham's vital signs were monitored and collected throughout the 16 minute flight, and used to develop life support systems for later human astronauts.[14]
Animal testing in space continues currently, with mice, ants, and other animals regularly being sent to the International Space Station.[15] In 2014, eight ant colonies were sent to the ISS to investigate the group behavior of ants in microgravity. The ISS allows for the investigation of animal behavior without sending them in specifically designed capsules.[15]
North American X-15 edit
Rocket-powered aircraft North American X-15 provided an early opportunity to study the effects of a near-space environment on human physiology.[16] At its highest operational speed and altitude, the X-15 provided approximately five minutes of weightlessness. This opportunity allowed for the development of devices to facilitate working in low pressure, high acceleration environments such as pressure suits, and telemetering systems to collect physiological data.[17] This data and technologies allowed for better mission planning for future space missions.[17]
Project Mercury edit
Space medicine was a critical factor in the United States human space program, starting with Project Mercury.[18] The main precaution taken by Mercury astronauts to defend against high G environments like launch and reentry was a couch with seat belts to make sure astronauts were not forcibly moved from their position. Additionally, experienced pilots proved to be better able to cope with high G scenarios.[11] One of the pressing concerns with Project Mercury's mission environment was the isolated nature of the cabin. There were deeper concerns about psychological issues than there were about physiological health effects. Substantial animal testing proved beyond a reasonable doubt to NASA engineers that spaceflight could be done safely provided a climate controlled environment.[11]
Project Gemini edit
The Gemini program primarily addressed the psychological issues from isolation in space with two crewmembers. Upon returning from space, it was recorded that crewmembers experienced a loss of balance and a decrease in anaerobic ability.[19]
Project Apollo edit
The Apollo program began with a substantial basis of medical knowledge and precautions from both Mercury and Gemini. The understanding of high and low G environments was well documented and the effects of isolation had been addressed with Gemini and Apollo having multiple occupants in one capsule. The primary research of the Apollo Program focused on pre-flight and post-flight monitoring.[19] Some Apollo mission plans were postponed or altered due to some or all crewmembers contracting a communicable disease. Apollo 14 instituted a form of quarantine for crewmembers so as to curb the passing of typical illnesses.[19] While the efficacy of the Flight Crew Health Stabilization Program was questionable as some crewmembers still contracted diseases,[19] the program showed enough results to maintain implementation with current space programs.[20]
Effects of space-travel edit
In October 2018, NASA-funded researchers found that lengthy journeys into outer space, including travel to the planet Mars, may substantially damage the gastrointestinal tissues of astronauts. The studies support earlier work that found such journeys could significantly damage the brains of astronauts, and age them prematurely.[21]
In November 2019, researchers reported that astronauts experienced serious blood flow and clot problems while on board the International Space Station, based on a six-month study of 11 healthy astronauts. The results may influence long-term spaceflight, including a mission to the planet Mars, according to the researchers.[22][23]
Blood clots edit
Deep vein thrombosis of the internal jugular vein of the neck was first discovered in 2020 in an astronaut on a long duration stay on the ISS, requiring treatment with blood thinners.[24] A subsequent study of eleven astronauts found slowed blood flow in the neck veins and even reversal of blood flow in two of the astronauts.[25] NASA is currently conducting more research to study whether these abnormalities could predispose astronauts to blood clots.
Cardiac rhythms edit
Heart rhythm disturbances have been seen among astronauts.[26] Most of these have been related to cardiovascular disease, but it is not clear whether this was due to pre-existing conditions or effects of space flight. It is hoped that advanced screening for coronary disease has greatly mitigated this risk. Other heart rhythm problems, such as atrial fibrillation, can develop over time, necessitating periodic screening of crewmembers’ heart rhythms. Beyond these terrestrial heart risks, some concern exists that prolonged exposure to microgravity may lead to heart rhythm disturbances. Although this has not been observed to date, further surveillance is warranted.
Decompression illness in spaceflight edit
In space, astronauts use a space suit, essentially a self-contained individual spacecraft, to do spacewalks, or extra-vehicular activities (EVAs). Spacesuits are generally inflated with 100% oxygen at a total pressure that is less than a third of normal atmospheric pressure. Eliminating inert atmospheric components such as nitrogen allows the astronaut to breathe comfortably, but also have the mobility to use their hands, arms, and legs to complete required work, which would be more difficult in a higher pressure suit.
After the astronaut dons the spacesuit, air is replaced by 100% oxygen in a process called a "nitrogen purge". In order to reduce the risk of decompression sickness, the astronaut must spend several hours "pre-breathing" at an intermediate nitrogen partial pressure, in order to let their body tissues outgas nitrogen slowly enough that bubbles are not formed. When the astronaut returns to the "shirt sleeve" environment of the spacecraft after an EVA, pressure is restored to whatever the operating pressure of that spacecraft may be, generally normal atmospheric pressure. Decompression illness in spaceflight consists of decompression sickness (DCS) and other injuries due to uncompensated changes in pressure, or barotrauma.
Decompression sickness edit
Decompression sickness is the injury to the tissues of the body resulting from the presence of nitrogen bubbles in the tissues and blood. This occurs due to a rapid reduction in ambient pressure causing the dissolved nitrogen to come out of solution as gas bubbles within the body.[27] In space the risk of DCS is significantly reduced by using a technique to wash out the nitrogen in the body's tissues. This is achieved by breathing 100% oxygen for a specified period of time before donning the spacesuit, and is continued after a nitrogen purge.[28][29] DCS may result from inadequate or interrupted pre-oxygenation time, or other factors including the astronaut's level of hydration, physical conditioning, prior injuries and age. Other risks of DCS include inadequate nitrogen purge in the EMU, a strenuous or excessively prolonged EVA, or a loss of suit pressure. Non-EVA crewmembers may also be at risk for DCS if there is a loss of spacecraft cabin pressure.
Symptoms of DCS in space may include chest pain, shortness of breath, cough or pain with a deep breath, unusual fatigue, lightheadedness, dizziness, headache, unexplained musculoskeletal pain, tingling or numbness, extremities weakness, or visual abnormalities.[30]
Primary treatment principles consist of in-suit repressurization to re-dissolve nitrogen bubbles,[31] 100% oxygen to re-oxygenate tissues,[32] and hydration to improve the circulation to injured tissues.[33]
Barotrauma edit
Barotrauma is the injury to the tissues of air filled spaces in the body as a result of differences in pressure between the body spaces and the ambient atmospheric pressure. Air filled spaces include the middle ears, paranasal sinuses, lungs and gastrointestinal tract.[34][35] One would be predisposed by a pre-existing upper respiratory infection, nasal allergies, recurrent changing pressures, dehydration, or a poor equalizing technique.
Positive pressure in the air filled spaces results from reduced barometric pressure during the depressurization phase of an EVA.[36][37] It can cause abdominal distension, ear or sinus pain, decreased hearing, and dental or jaw pain.[35][38] Abdominal distension can be treated with extending the abdomen, gentle massage and encourage passing flatus. Ear and sinus pressure can be relieved with passive release of positive pressure.[39] Pretreatment for susceptible individuals can include oral and nasal decongestants, or oral and nasal steroids.[40]
Negative pressure in air fill spaces results from increased barometric pressure during repressurization after an EVA or following a planned restoration of a reduced cabin pressure. Common symptoms include ear or sinus pain, decreased hearing, and tooth or jaw pain.[41]
Treatment may include active positive pressure equalization of ears and sinuses,[42][39] oral and nasal decongestants, or oral and nasal steroids, and appropriate pain medication if needed.[40]
Decreased immune system functioning edit
Astronauts in space have weakened immune systems, which means that in addition to increased vulnerability to new exposures, viruses already present in the body—which would normally be suppressed—become active.[43] In space, T-cells do not reproduce properly, and the cells that do exist are less able to fight off infection.[44] NASA research is measuring the change in the immune systems of its astronauts as well as performing experiments with T-cells in space.
On April 29, 2013, scientists in Rensselaer Polytechnic Institute, funded by NASA, reported that, during spaceflight on the International Space Station, microbes seem to adapt to the space environment in ways "not observed on Earth" and in ways that "can lead to increases in growth and virulence".[45]
In March 2019, NASA reported that latent viruses in humans may be activated during space missions, adding possibly more risk to astronauts in future deep-space missions.[46]
Increased infection risk edit
A 2006 Space Shuttle experiment found that Salmonella typhimurium, a bacterium that can cause food poisoning, became more virulent when cultivated in space.[47] On April 29, 2013, scientists in Rensselaer Polytechnic Institute, funded by NASA, reported that, during spaceflight on the International Space Station, microbes seem to adapt to the space environment in ways "not observed on Earth" and in ways that "can lead to increases in growth and virulence".[45] More recently, in 2017, bacteria were found to be more resistant to antibiotics and to thrive in the near-weightlessness of space.[48] Microorganisms have been observed to survive the vacuum of outer space.[49][50] Researchers in 2018 reported, after detecting the presence on the International Space Station (ISS) of five Enterobacter bugandensis bacterial strains, none pathogenic to humans, that microorganisms on ISS should be carefully monitored to continue assuring a medically healthy environment for astronauts.[51][52]
Effects of fatigue edit
Human spaceflight often requires astronaut crews to endure long periods without rest. Studies have shown that lack of sleep can cause fatigue that leads to errors while performing critical tasks.[53][54][55] Also, individuals who are fatigued often cannot determine the degree of their impairment.[56] Astronauts and ground crews frequently suffer from the effects of sleep deprivation and circadian rhythm disruption. Fatigue due to sleep loss, sleep shifting and work overload could cause performance errors that put space flight participants at risk of compromising mission objectives as well as the health and safety of those on board.
Loss of balance edit
Leaving and returning to Earth's gravity causes “space sickness,” dizziness, and loss of balance in astronauts. By studying how changes can affect balance in the human body—involving the senses, the brain, the inner ear, and blood pressure—NASA hopes to develop treatments that can be used on Earth and in space to correct balance disorders. Until then, NASA's astronauts must rely on a medication called Midodrine (an “anti-dizzy” pill that temporarily increases blood pressure), and/or promethazine to help carry out the tasks they need to do to return home safely.[57]
Loss of bone density edit
Spaceflight osteopenia is the bone loss associated with human spaceflight.[3] The metabolism of calcium is limited in microgravity and will cause calcium to leak out of bones.[10] After a 3–4 month trip into space, it takes about 2–3 years to regain lost bone density.[58][59] New techniques are being developed to help astronauts recover faster. Research in the following areas holds the potential to aid the process of growing new bone:
- Diet and Exercise changes may reduce osteoporosis.
- Vibration Therapy may stimulate bone growth.[60]
- Medication could trigger the body to produce more of the protein responsible for bone growth and formation.
Loss of muscle mass edit
In space, muscles in the legs, back, spine, and heart weaken and waste away because they no longer are needed to overcome gravity, just as people lose muscle when they age due to reduced physical activity.[3] Astronauts rely on research in the following areas to build muscle and maintain body mass:
- Exercise may build muscle if at least two hours a day is spent doing resistance training routines.
- Neuromuscular Electrical Stimulation as a method to prevent muscle atrophy.[17]
Impairment of eyesight edit
During long space flight missions, astronauts may develop ocular changes and visual impairment collectively known as the Space Associated Neuro-ocular Syndrome (SANS).[2][3][61][62][63][64][65][66] Such vision problems may be a major concern for future deep space flight missions, including a human mission to Mars.[61][62][63][64][67]
Loss of mental abilities and risk of Alzheimer's disease edit
On December 31, 2012, a NASA-supported study reported that human spaceflight may harm the brain of astronauts and accelerate the onset of Alzheimer's disease.[68][69][70]
On 2 November 2017, scientists reported that significant changes in the position and structure of the brain have been found in astronauts who have taken trips in space, based on MRI studies. Astronauts who took longer space trips were associated with greater brain changes.[71][72]
Orthostatic intolerance edit
"Under the effects of the earth's gravity, blood and other body fluids are pulled towards the lower body. When gravity is taken away or reduced during space exploration, the blood tends to collect in the upper body instead, resulting in facial edema and other unwelcome side effects. Upon return to earth, the blood begins to pool in the lower extremities again, resulting in orthostatic hypotension."[74]
In space, astronauts lose fluid volume—including up to 22% of their blood volume. Because it has less blood to pump, the heart will atrophy. A weakened heart results in low blood pressure and can produce a problem with “orthostatic tolerance,” or the body's ability to send enough oxygen to the brain without fainting or becoming dizzy.[74]
Radiation effects edit
Soviet cosmonaut Valentin Lebedev, who spent 211 days in orbit during 1982 (an absolute record for stay in Earth's orbit), lost his eyesight to progressive cataract. Lebedev stated: “I suffered from a lot of radiation in space. It was all concealed back then, during the Soviet years, but now I can say that I caused damage to my health because of that flight.”[3][79] On 31 May 2013, NASA scientists reported that a possible human mission to Mars may involve a great radiation risk based on the amount of energetic particle radiation detected by the RAD on the Mars Science Laboratory while traveling from the Earth to Mars in 2011–2012.[67][75][76][77][78]
Sleep disorders edit
Spaceflight has been observed to disrupt physiological processes that influence sleep patterns in human beings.[80] Astronauts exhibit asynchronized cortisol rhythmicity, dampened diurnal fluctuations in body temperature, and diminished sleep quality.[80] Sleep pattern disruption in astronauts is a form of extrinsic (environmentally caused) circadian rhythm sleep disorder.[80]
Spaceflight analogues edit
Biomedical research in space is expensive and logistically and technically complicated, and thus limited. Conducting medical research in space alone will not provide humans with the depth of knowledge needed to ensure the safety of inter-planetary travelers. Complementary to research in space is the use of spaceflight analogues. Analogues are particularly useful for the study of immunity, sleep, psychological factors, human performance, habitability, and telemedicine. Examples of spaceflight analogues include confinement chambers (Mars-500), sub-aqua habitats (NEEMO), and Antarctic (Concordia Station) and Arctic FMARS and (Haughton–Mars Project) stations.[67]
Space medicine careers edit
Physicians in space medicine generally work in operations or research at NASA or, more recently, space companies that are flying private or commercial astronauts or spaceflight participants.
Operational space medicine provides direct medical support to astronauts and spaceflight participants, conducting medical screening and overseeing their preflight, inflight, and postflight medical care and preparations. As such, operational space medicine physicians are generally physicians trained in a clinical specialty--such as emergency medicine, family medicine, or internal medicine--who have undergone additional residency or fellowship training in aerospace medicine. Board certification in aerospace medicine is the gold standard for physicians who practice operational space medicine.
Research physicians study specific space medical problems, such as the Space Associated Neuro-ocular Syndrome, or focus on medical capabilities for future deep space exploration missions. Research physicians do not have clinical responsibilities in the care of astronauts and thereby are often not specialty-trained in aerospace medicine.
Related degrees, areas of specialization, and certifications edit
- Aeromedical certification
- Aerospace medicine
- Aerospace studies
- Occupational and preventive medicine
- Global Health
- Public Health
- Disaster medicine
- Prehospital medicine
- Wilderness and extreme medicine
Space nursing edit
Space nursing is the nursing specialty that studies how space travel impacts human response patterns. Similar to space medicine, the specialty also contributes to knowledge about nursing care of earthbound patients.[81][82]
Medicine in flight edit
Sleep medicine edit
The use of hypnotic sleep aids is widespread among astronauts, with one 10 year long study finding that 75% and 78% of ISS and space shuttle crew members reported taking such medications while in space.[83] Of astronauts who took hypnotic medications, frequency of use was 52% of all nights. NASA allocates 8.5 hours of 'downtime' for sleep per day for astronauts aboard the ISS, but the average duration of sleep is only 6 hours.[84] Poor sleep quality and quantity can compromise the daytime performance and attentiveness of space crew. As such, improving nighttime sleep has been a topic of NASA-funded research for more than half a century.[85] The following pharmacological and environmental strategies have been investigated in the context of sleep in space:
- Light therapy, involving exposure to visible light at varying intensities and wavelengths to entrain circadian rhythm, is key a topic of interest in NASA-funded research.[86] Various photoreceptors in the human eye such as melanopsin, rhodopsin, and photopsin communicate with the suprachiasmatic nucleus (the master circadian pacemaker of the brain) to entrain circadian rhythm.[87] Melanopsin photoreceptors are most sensitive to blue light wavelengths in the range of 470-490 nm (blue light).[88] NASA has trialed and implemented rhythmic light panels on the ISS to assist entrain the circadian rhythms of astronauts.[89][90] NASA is soon to test more advanced light panels that change their output light intensity and wavelengths according to time of day, with red-tinted lights (<600 nm) set to be used at night to provide visibility at 'night' and shorter wavelengths of high light intensity to be used in the 'morning' or at times where alertness and vigilance are needed.[91][92]
- Melatonin, a naturally occurring hormone secreted by pineal gland, has shown positive effects in reducing sleep latency in orbit.[93]
- Nonbenzodiazepines sedative-hypnotics (also known as "z drugs") such as Zolpidem Zopiclone, and Zaleplon are the most frequently used medications on shuttle missions. Despite their widespread use amongst astronauts, relatively little research has been conducted on nonbenzodiazepines in the context of spaceflight. In earthling contexts, nonbenzodiazepines have been shown to produce less residual impairment than benzodiazepines.[94] The shortest acting nonbenzodiazepine, Zaleplon, produces little to no cognitive impairment (at clinically relevant doses) upon waking even when dosed as little as hour before awakening.[95] Research indicates that astronauts frequently take second doses of hypnotic drugs, the shorter duration of action of nonbenzodiazepines have been implicated as being preferable for such situations.[96]
- Benzodiazepines are frequently used medications in space, though less often than nonbenzodiazepine "z-drugs".[97] The long acting nature of benzodiazepines used by astronauts, such as temazepam, have been labelled "non-ideal" for spaceflight use due to a high tendency of causing morning impairments.[96]
- Modafinil, a wakefulness drug, is available on the space station to mitigate the deleterious effects of sleep disruption and "optimise performance while fatigued".[98] Modafinil has shown positive results in restoring cognitive function to baseline in the face of total sleep deprivation, though no studies examining modafinil's effects specifically in astronauts have been conducted
Ultrasound and space edit
Ultrasound is the main diagnostic imaging tool on ISS and for the foreseeable future missions. X-rays and CT scans involve radiation which is unacceptable in the space environment. Though MRI uses magnetics to create images, it is too large at present to consider as a viable option. Ultrasound, which uses sound waves to create images and comes in laptop size packages, provides imaging of a wide variety of tissues and organs. It is currently being used to look at the eyeball and the optic nerve to help determine the cause(s) of changes that NASA has noted mostly in long duration astronauts. NASA is also pushing the limits of ultrasound use regarding musculoskeletal problems as these are some of the most common and most likely problems to occur. Significant challenges to using ultrasounds on space missions is training the astronaut to use the equipment (ultrasound technicians spend years in training and developing the skills necessary to be "good" at their job) as well as interpreting the images that are captured. Much of ultrasound interpretation is done real-time but it is impractical to train astronauts to actually read/interpret ultrasounds. Thus, the data is currently being sent back to mission control and forwarded to medical personnel to read and interpret. Future exploration class missions will need to be autonomous due to transmission times taking too long for urgent/emergent medical conditions. The ability to be autonomous, or to use other equipment such as MRIs, is currently being researched.
Space Shuttle era edit
With the additional lifting capability presented by the Space Shuttle program, NASA designers were able to create a more comprehensive medical readiness kit. The SOMS consists of two separate packages: the Medications and Bandage Kit (MBK) and the Emergency Medical Kit (EMK). While the MBK contained capsulate medications (tablets, capsules, and suppositories), bandage materials, and topical medication, the EMK had medications to be administered by injection, items for performing minor surgeries, diagnostic/therapeutic items, and a microbiological test kit.[99]
John Glenn, the first American astronaut to orbit the Earth, returned with much fanfare to space once again on STS-95 at 77 years of age to confront the physiological challenges preventing long-term space travel for astronauts—loss of bone density, loss of muscle mass, balance disorders, sleep disturbances, cardiovascular changes, and immune system depression—all of which are problems confronting aging people as well as astronauts.[100]
Future investigations edit
Feasibility of Long Duration Space Flights edit
In the interest of creating the possibility of longer duration space flight, NASA has invested in the research and application of preventative space medicine, not only for medically preventable pathologies but trauma as well. Although trauma constitutes more of a life-threatening situation, medically preventable pathologies pose more of a threat to astronauts. "The involved crewmember is endangered because of mission stress and the lack of complete treatment capabilities on board the spacecraft, which could result in the manifestation of more severe symptoms than those usually associated with the same disease in the terrestrial environment. Also, the situation is potentially hazardous for the other crewmembers because the small, closed, ecological system of the spacecraft is conducive to disease transmission. Even if the disease is not transmitted, the safety of the other crewmembers may be jeopardized by the loss of the capabilities of the crewmember who is ill. Such an occurrence will be more serious and potentially hazardous as the durations of crewed missions increase and as operational procedures become more complex. Not only do the health and safety of the crewmembers become critical, but the probability of mission success is lessened if the illness occurs during flight. Aborting a mission to return an ill crewmember before mission goals are completed is costly and potentially dangerous."[101] Treatment of trauma may involve surgery in zero-gravity,[102] which is a challenging proposition given the need for blood sample containment. Diagnosis and monitoring of crew members is a particularly vital need. NASA tested the rHEALTH ONE[103] to advance this capability for on-orbit, travel to Moon and Mars. This capability is mapped to Risk of Adverse Health Outcomes and Decrements in Performance Due to Medical Conditions that occur in Mission, as well as Long Term Health Outcomes Due to Mission Exposures. Without an approach to perform onboard medical monitoring, loss of crew members may jeopardize long duration missions.
Impact on science and medicine edit
Astronauts are not the only ones who benefit from space medicine research. Several medical products have been developed that are space spinoffs, which are practical applications for the field of medicine arising out of the space program. Because of joint research efforts between NASA, the National Institutes on Aging (a part of the National Institutes of Health), and other aging-related organizations, space exploration has benefited a particular segment of society, seniors. Evidence of aging related medical research conducted in space was most publicly noticeable during STS-95. These spin-offs are sometimes termed as "exomedicine".
Pre-Mercury through Apollo edit
- Radiation therapy for the treatment of cancer: In conjunction with the Cleveland Clinic, the cyclotron at Glenn Research Center in Cleveland, Ohio was used in the first clinical trials for the treatment and evaluation of neutron therapy for cancer patients.[104]
- Foldable walkers: Made from a lightweight metal material developed by NASA for aircraft and spacecraft, foldable walkers are portable and easy to manage.
- Personal alert systems: These are emergency alert devices that can be worn by individuals who may require emergency medical or safety assistance. When a button is pushed, the device sends a signal to a remote location for help. To send the signal, the device relies on telemetry technology developed at NASA.
- CAT and MRI scans: These devices are used by hospitals to see inside the human body. Their development would not have been possible without the technology provided by NASA after it found a way to take better pictures of the Earth's moon.[105]
- Neuromuscular Electric Stimulation (NMES): A form of treatment originally developed to combat muscle atrophy in space that has been found to have applications outside of space. A prominent example of NMES being used outside of space medicine is muscle stimulator devices for paralyzed individuals. These devices can be used from up to half an hour per day to prevent muscle atrophy in paralyzed individuals.[106] It provides electrical stimulation to muscles which is equal to jogging three miles per week. A well-known example is that Christopher Reeve used these in his therapy. Outside of paralyzed individuals, it also has applications in sports medicine, where it is used to manage or prevent potential damages that those high-intensity lifestyles have on athletes.[107]
- Orthopedic evaluation tools: equipment to evaluate posture, gait and balance disturbances was developed at NASA, along with a radiation-free way to measure bone flexibility using vibration.
- Diabetic foot mapping: This technique was developed at NASA's center in Cleveland, Ohio to help monitor the effects of diabetes in feet.
- Foam cushioning: special foam used for cushioning astronauts during liftoff is used in pillows and mattresses at many nursing homes and hospitals to help prevent ulcers, relieve pressure, and provide a better night's sleep.
- Kidney dialysis machines: the Marquardt Corporation, an ancestor company with NASA, were developing a system that would purify and recycle water during space missions in the late 1960s.[108] From this project, the Marquardt Corporation observed that these processes could be used in removing toxic waste from used dialysis fluid.[108] This allowed the development of a kidney dialysis machine.[108] These machines rely on technology developed by NASA in order to process and remove toxic waste from used dialysis fluid.[108]
- Talking wheelchairs: paralyzed individuals who have difficulty speaking may use a talking feature on their wheelchairs which was developed by NASA to create synthesized speech for aircraft. "Talking Wheelchairs" or The Versatile Portable Speech Prosthesis (VSP) is a technology that aids in the communication for non-verbal persons.[110] The project started in May 1978 and finished in November 1981.[110] Originally, this technology was created for people who were diagnosed with cerebral palsy who were using traditional electric wheelchairs.[110] This technology is portable and versatile, as well as a highly successful speech prosthesis.[110] However, the nickname "talking wheelchair" has created some separation from the wheelchair itself.[110] The VSP is easily accessible to the person using it by operation of single or multiple switches or by keyboard, and uses a synthetic voice used for verbal speech.[110] The synthetic voice provides communication opportunities that regular speaking persons have such as: communicating with people in a crowd, communicating in the dark, communicating with people who have vision problems, communicating with younger children, communicating when the listener's back is turned, etc.[110] The synthetic voice also provides a sense of personal and individual communication as the keyboard can be programmed with “fun” words as well as “throw-away lines”.[110] The first version of the versatile portable speech prosthesis was completed in May 1979.[110] There were additions made to the VSP in November 1979 and provided more controls for speech.[110] By November 1979, VSP was capable of taking English text and successful in putting out English speech.[110] The user was also able to store and retrieve vocabulary, as well as edit and create new vocabulary.[110] The controls and plugs on the VSP were versatile allowing plug-and-go ability.[110] With the limitations of ASR systems, Portable Speech Prosthesis have moved to the use of Silent Speech Recognition (SSR).[111] The goal of using SSR with VSP is to recognize information that is speech related with some modals such as surface electromyography (sEMG).[111] Speech recognition models used algorithms for extracting speech-related features through the sEMG signals.[111] The patterns of sEMG signals used grammar models to recognize sequences of words.[111] Phoneme-based models were also used when recognizing vocabulary of previously untrained words.[111] Multi-point sensors were used with these algorithms in which they could be arranged in a flexible way to record the measurements of sEMG signals from the small articular muscles found in the human face and neck.[111]
- Collapsible, lightweight wheelchairs: wheelchairs designed for portability that can be folded and put into trunks of cars. They rely on synthetic materials that NASA developed for its air and space craft
- Surgically implantable heart pacemaker: these devices depend on technologies developed by NASA for use with satellites. They communicate information about the activity of the pacemaker, such as how much time remains before the batteries need to be replaced.[112]
- Implantable heart defibrillator: this tool continuously monitors heart activity and can deliver an electric shock to restore heartbeat regularity.
- EMS communications: technology used to communicate telemetry between Earth and space was developed by NASA to monitor the health of astronauts in space from the ground. Ambulances use this same technology to send information—like EKG readings—from patients in transport to hospitals. This allows faster and better treatment.
- Weightlessness therapy: The weightlessness of space can allow some individuals with limited mobility on Earth—even those normally confined to wheelchairs—the freedom to move about with ease. Physicist Stephen Hawking took advantage of weightlessness in NASA's Vomit Comet aircraft in 2007.[113] This idea also led to the development of the Anti-Gravity Treadmill from NASA technology, which employs "differential air pressure to mimic...gravity".[114]
Ultrasound microgravity edit
The Advanced Diagnostic Ultrasound in Microgravity Study is funded by the National Space Biomedical Research Institute and involves the use of ultrasound among Astronauts including former ISS Commanders Leroy Chiao and Gennady Padalka who are guided by remote experts to diagnose and potentially treat hundreds of medical conditions in space. This study has a widespread impact and has been extended to cover professional and Olympic sports injuries as well as medical students. It is anticipated that remote guided ultrasound will have application on Earth in emergency and rural care situations. Findings from this study were submitted for publication to the journal Radiology aboard the International Space Station; the first article submitted in space.[115][116][117]
See also edit
- Artificial gravity
- Aviation medicine
- Bioastronautics
- Effect of spaceflight on the human body
- Fatigue and sleep loss during spaceflight
- Intervertebral disc damage and spaceflight
- List of microorganisms tested in outer space
- Mars analog habitat
- Medical treatment during spaceflight
- Microgravity University
- Reduced-gravity aircraft
- Renal stone formation in space
- Spaceflight osteopenia
- Spaceflight radiation carcinogenesis
- Space food
- Space nursing
- Space Nursing Society
- Space pharmacology
- Team composition and cohesion in spaceflight missions
- Visual impairment due to intracranial pressure
References edit
- Notes
- ^ "International Space Station Medical Monitoring (ISS Medical Monitoring)". 3 December 2013. Retrieved January 13, 2014.
- ^ a b Chang, Kenneth (January 27, 2014). "Beings Not Made for Space". New York Times. Retrieved January 27, 2014.
- ^ a b c d e Mann, Adam (July 23, 2012). "Blindness, Bone Loss, and Space Farts: Astronaut Medical Oddities". Wired. Retrieved July 23, 2012.
- ^ Dunn, Marcia (October 29, 2015). "Report: NASA needs better handle on health hazards for Mars". AP News. Retrieved October 30, 2015.
- ^ Staff (October 29, 2015). "NASA's Efforts to Manage Health and Human Performance Risks for Space Exploration (IG-16-003)" (PDF). NASA. Retrieved October 29, 2015.
- ^ Andrew Walker (21 November 2005). "Project Paperclip: Dark side of the Moon". BBC News. Retrieved 2012-04-25.
- ^ "Former Nazi removed from Space Hall of Fame". NBC News. Associated Press. 2006-05-19. Retrieved 2006-05-19.
- ^ "Russian Space Medicine". Russian Military. 21 July 2021. Retrieved 2021-07-28.
- ^ "Soviet Space Medicine Video History Collection, 1989". Smithsonian Institution. 20 July 2021. Retrieved 2021-07-29.
- ^ a b c Herald of the Russian Academy of Sciences. Pleiades Publishing Ltd. 2013. doi:10.1134/11480.1555-6492.
- ^ a b c d e "This New Ocean - Ch2-3". history.nasa.gov. Retrieved 2022-03-11.
- ^ a b "Animals in Space". history.nasa.gov. Retrieved 2022-04-15.
- ^ "This New Ocean - Ch10-3". history.nasa.gov. Retrieved 2022-04-15.
- ^ a b "October Flashback - National Geographic Magazine". 2007-11-12. Archived from the original on 2007-11-12. Retrieved 2022-04-15.
- ^ a b "Focus on animal experiments onboard of the ISS". Recherche animale. Retrieved 2022-04-29.
- ^ "This New Ocean - Ch2-3". www.hq.nasa.gov. Retrieved 2022-03-11.
- ^ a b c "This New Ocean - Ch2-2". history.nasa.gov. Retrieved 2022-03-11.
- ^ Link, Mae Mills (1965). Space Medicine in Project Mercury (NASA Special Publication). NASA SP (Series). Washington, D.C.: Scientific and Technical Information Office, National Aeronautics and Space Administration. OCLC 1084318604. NASA SP-4003. Retrieved 17 February 2019.
- ^ a b c d Johnston, Richard S.; Dietlein, Lawrence F.; Berry, Charles A. (1975). Biomedical results of Apollo. Scientific and Technical Information Office, National Aeronautics and Space Administration. OCLC 1222824163.
- ^ "Astronauts Enter Quarantine for Upcoming Crew-1 Mission – Commercial Crew Program". blogs.nasa.gov. Retrieved 2022-04-29.
- ^ Griffin, Andrew (2 October 2018). "Travelling to Mars and deep into space could kill astronauts by destroying their guts, finds Nasa-funded study – Previous work has shown that astronauts could age prematurely and have damaged brain tissue after long journeys". The Independent. Archived from the original on 2022-05-24. Retrieved 2 October 2018.
- ^ Strickland, Ashley (15 November 2019). "Astronauts experienced reverse blood flow and blood clots on the space station, study says". CNN News. Retrieved 22 November 2019.
- ^ Marshall-Goebel, Karina; et al. (13 November 2019). "Assessment of Jugular Venous Blood Flow Stasis and Thrombosis During Spaceflight". JAMA Network Open. 2 (11): e1915011. doi:10.1001/jamanetworkopen.2019.15011. PMC 6902784. PMID 31722025.
- ^ David, Leonard (2020-01-03). "An Astronaut Got a Blood Clot in Space. Here's How Doctors on Earth Fixed It". Space.com. Retrieved 2023-12-30.
- ^ Care, University of North Carolina Health. "Blood clot expert working with NASA to study blood flow, clot formation in zero gravity". phys.org. Retrieved 2023-12-30.
- ^ Platts, S. H., Stenger, M. B., Phillips, T. R., Brown, A. K., Arzeno, N. M., Levine, B., & Summers, R. (2009). Evidence Based Review: Risk of Cardiac Rhythm Problems During Spaceflight.
- ^ Ackles, KN (1973). "Blood-Bubble Interaction in Decompression Sickness". Defence R&D Canada (DRDC) Technical Report. DCIEM-73–CP-960. Archived from the original on August 21, 2009. Retrieved 23 May 2010.
{{cite journal}}
: CS1 maint: unfit URL (link) - ^ Nevills, Amiko (2006). "Preflight Interview: Joe Tanner". NASA. Archived from the original on 12 May 2013. Retrieved 26 June 2010.
- ^ Webb, James T; Olson, RM; Krutz, RW; Dixon, G; Barnicott, PT (1989). "Human tolerance to 100% oxygen at 9.5 psia during five daily simulated 8-hour EVA exposures". Aviation, Space, and Environmental Medicine. 60 (5): 415–21. doi:10.4271/881071. PMID 2730484.
- ^ Francis, T James R; Mitchell, Simon J (2003). "10.6: Manifestations of Decompression Disorders". In Brubakk, Alf O; Neuman, Tom S (eds.). Bennett and Elliott's physiology and medicine of diving (5th Revised ed.). United States: Saunders. pp. 578–584. ISBN 978-0-7020-2571-6. OCLC 51607923.
- ^ Berghage, Thomas E; Vorosmarti Jr, James; Barnard, EEP (1978). "Recompression treatment tables used throughout the world by government and industry". US Naval Medical Research Center Technical Report. NMRI-78-16. Archived from the original on August 5, 2009. Retrieved 25 May 2010.
{{cite journal}}
: CS1 maint: unfit URL (link) - ^ Marx, John (2010). Rosen's emergency medicine: concepts and clinical practice (7th ed.). Philadelphia, PA: Mosby/Elsevier. ISBN 978-0-323-05472-0.
- ^ Thalmann, Edward D (March–April 2004). "Decompression Illness: What Is It and What Is The Treatment?". Divers Alert Network. Archived from the original on 13 June 2010. Retrieved 3 August 2010.
- ^ US Navy Diving Manual, 6th revision. United States: US Naval Sea Systems Command. 2006. Archived from the original on 2008-05-02. Retrieved 2008-05-26.
- ^ a b Brubakk, A. O.; Neuman, T. S. (2003). Bennett and Elliott's physiology and medicine of diving, 5th Rev ed. United States: Saunders Ltd. p. 800. ISBN 978-0-7020-2571-6.
- ^ Vogt L. (1991). "European EVA decompression sickness risks". Acta Astronautica. 23. Wenzel J., Skoog A. I., Luck S., Svensson B.: 195–205. Bibcode:1991AcAau..23..195V. doi:10.1016/0094-5765(91)90119-p. PMID 11537125.
- ^ Newman, D., & Barrat, M. (1997). Life support and performance issues for extravehicular activity (EVA). Fundamentals of Space Life Sciences, 2.
- ^ Robichaud, R.; McNally, M. E. (January 2005). "Barodontalgia as a differential diagnosis: symptoms and findings". Journal of the Canadian Dental Association. 71 (1): 39–42. PMID 15649340. Retrieved 2008-07-19.
- ^ a b Kay, E (2000). "Prevention of middle ear barotrauma". Doc's Diving Medicine. staff.washington.edu. Archived from the original on 16 January 2017. Retrieved 13 January 2017.
- ^ a b Kaplan, Joseph. Alcock, Joe (ed.). "Barotrauma Medication". emedicine.medscape.com. Retrieved 15 January 2017.
- ^ Clark, J. B. (2008). Decompression-related disorders: pressurization systems, barotrauma, and altitude sickness. In Principles of Clinical Medicine for Space Flight (pp. 247–271). Springer, New York, NY.
- ^ Hidir Y. (2011). "A comparative study on efficiency of middle ear pressure equalization techniques in healthy volunteers". Auris Nasus Larynx. 38 (4). Ulus S., Karahatay S., Satar B.: 450–455. doi:10.1016/j.anl.2010.11.014. PMID 21216116.
- ^ Pierson D.L. (2005). "Epstein–Barr virus shedding by astronauts during space flight". Brain, Behavior, and Immunity. 19 (3). Stowe R.P., Phillips T.M., Lugg D.J., Mehta S.K.: 235–242. doi:10.1016/j.bbi.2004.08.001. PMID 15797312. S2CID 24367925.
- ^ Cogoli A (1996). "Gravitational physiology of human immune cells: a review of in vivo, ex vivo and in vitro studies". Journal of Gravitational Physiology. 3 (1): 1–9. PMID 11539302.
- ^ a b Kim W, et al. (April 29, 2013). "Spaceflight Promotes Biofilm Formation by Pseudomonas aeruginosa". PLOS ONE. 8 (4): e6237. Bibcode:2013PLoSO...862437K. doi:10.1371/journal.pone.0062437. PMC 3639165. PMID 23658630.
- ^ Staff (15 March 2019). "Dormant viruses activate during spaceflight -- NASA investigates - The stress of spaceflight gives viruses a holiday from immune surveillance, putting future deep-space missions in jeopardy". EurekAlert!. Retrieved 16 March 2019.
- ^ Caspermeyer, Joe (23 September 2007). "Space flight shown to alter ability of bacteria to cause disease". Arizona State University. Archived from the original on 14 September 2017. Retrieved 14 September 2017.
- ^ Dvorsky, George (13 September 2017). "Alarming Study Indicates Why Certain Bacteria Are More Resistant to Drugs in Space". Gizmodo. Retrieved 14 September 2017.
- ^ Dose, K.; Bieger-Dose, A.; Dillmann, R.; Gill, M.; Kerz, O.; Klein, A.; Meinert, H.; Nawroth, T.; Risi, S.; Stridde, C. (1995). "ERA-experiment "space biochemistry"". Advances in Space Research. 16 (8): 119–129. Bibcode:1995AdSpR..16h.119D. doi:10.1016/0273-1177(95)00280-R. PMID 11542696.
- ^ Horneck G.; Eschweiler, U.; Reitz, G.; Wehner, J.; Willimek, R.; Strauch, K. (1995). "Biological responses to space: results of the experiment "Exobiological Unit" of ERA on EURECA I". Adv. Space Res. 16 (8): 105–18. Bibcode:1995AdSpR..16h.105H. doi:10.1016/0273-1177(95)00279-N. PMID 11542695.
- ^ BioMed Central (22 November 2018). "ISS microbes should be monitored to avoid threat to astronaut health". EurekAlert!. Retrieved 25 November 2018.
- ^ Singh, Nitin K.; et al. (23 November 2018). "Multi-drug resistant Enterobacter bugandensis species isolated from the International Space Station and comparative genomic analyses with human pathogenic strains". BMC Microbiology. 18 (1): 175. doi:10.1186/s12866-018-1325-2. PMC 6251167. PMID 30466389.
- ^ Harrison, Yvonne; Horne, James (June 1998). "Sleep loss impairs short and novel language tasks having a prefrontal focus". Journal of Sleep Research. 7 (2): 95–100. doi:10.1046/j.1365-2869.1998.00104.x. PMID 9682180. S2CID 34980267.
- ^ Durmer, JS; Dinges, DF (Mar 2005). "Neurocognitive consequences of sleep deprivation" (PDF). Seminars in Neurology. 25 (1): 117–29. doi:10.1055/s-2005-867080. PMC 3564638. PMID 15798944. Archived from the original (PDF) on 2012-06-17.
- ^ Banks, S; Dinges, DF (15 August 2007). "Behavioral and physiological consequences of sleep restriction". Journal of Clinical Sleep Medicine. 3 (5): 519–28. doi:10.5664/jcsm.26918. PMC 1978335. PMID 17803017.
- ^ Whitmire, A.M.; Leveton, L.B; Barger, L.; Brainard, G.; Dinges, D.F.; Klerman, E.; Shea, C. "Risk of Performance Errors due to Sleep Loss, Circadian Desynchronization, Fatigue, and Work Overload" (PDF). Human Health and Performance Risks of Space Exploration Missions: Evidence reviewed by the NASA Human Research Program. p. 88. Retrieved 17 May 2012.
- ^ Shi S. J. (2011). "Effects of promethazine and midodrine on orthostatic tolerance". Aviation, Space, and Environmental Medicine. 82 (1). Platts S. H., Ziegler M. G., Meck J. V.: 9–12. doi:10.3357/asem.2888.2011. PMID 21235099.
- ^ Sibonga J. D. (2007). "Recovery of spaceflight-induced bone loss: bone mineral density after long-duration missions as fitted with an exponential function". Bone. 41 (6). Evans H. J., Sung H. G., Spector E. R., Lang T. F., Oganov V. S., LeBlanc A. D.: 973–978. doi:10.1016/j.bone.2007.08.022. hdl:2060/20070032016. PMID 17931994.
- ^ Williams D. (2009). "Acclimation during space flight: effects on human physiology". Canadian Medical Association Journal. 180 (13). Kuipers A., Mukai C., Thirsk R.: 1317–1323. doi:10.1503/cmaj.090628. PMC 2696527. PMID 19509005.
- ^ Hawkey A (2007). "Low magnitude, high frequency signals could reduce bone loss during spaceflight". Journal of the British Interplanetary Society. 60: 278–284. Bibcode:2007JBIS...60..278H.
- ^ a b Mader, T. H.; et al. (2011). "Optic Disc Edema, Globe Flattening, Choroidal Folds, and Hyperopic Shifts Observed in Astronauts after Long-duration Space Flight". Ophthalmology. 118 (10): 2058–2069. doi:10.1016/j.ophtha.2011.06.021. PMID 21849212. S2CID 13965518.
- ^ a b Puiu, Tibi (November 9, 2011). "Astronauts' vision severely affected during long space missions". zmescience.com. Retrieved February 9, 2012.
- ^ a b "Male Astronauts Return With Eye Problems (video)". CNN News. 9 Feb 2012. Retrieved 2012-04-25.
- ^ a b Space Staff (13 March 2012). "Spaceflight Bad for Astronauts' Vision, Study Suggests". Space.com. Retrieved 14 March 2012.
- ^ Kramer, Larry A.; et al. (13 March 2012). "Orbital and Intracranial Effects of Microgravity: Findings at 3-T MR Imaging". Radiology. 263 (3): 819–827. doi:10.1148/radiol.12111986. PMID 22416248.
- ^ Howell, Elizabeth (3 November 2017). "Brain Changes in Space Could Be Linked to Vision Problems in Astronauts". Seeker. Retrieved 3 November 2017.
- ^ a b c Fong, MD, Kevin (12 February 2014). "The Strange, Deadly Effects Mars Would Have on Your Body". Wired. Retrieved 12 February 2014.
- ^ Cherry, Jonathan D.; Frost, Jeffrey L.; Lemere, Cynthia A.; Williams, Jacqueline P.; Olschowka, John A.; O'Banion, M. Kerry (2012). "Galactic Cosmic Radiation Leads to Cognitive Impairment and Increased Aβ Plaque Accumulation in a Mouse Model of Alzheimer's Disease". PLOS ONE. 7 (12): e53275. Bibcode:2012PLoSO...753275C. doi:10.1371/journal.pone.0053275. PMC 3534034. PMID 23300905.
- ^ Staff (January 1, 2013). "Study Shows that Space Travel is Harmful to the Brain and Could Accelerate Onset of Alzheimer's". SpaceRef. Archived from the original on May 21, 2020. Retrieved January 7, 2013.
- ^ Cowing, Keith (January 3, 2013). "Important Research Results NASA Is Not Talking About (Update)". NASA Watch. Retrieved January 7, 2013.
- ^ Roberts, Donna R.; et al. (2 November 2017). "Effects of Spaceflight on Astronaut Brain Structure as Indicated on MRI". New England Journal of Medicine. 377 (18): 1746–1753. doi:10.1056/NEJMoa1705129. PMID 29091569. S2CID 205102116.
- ^ Foley, Katherine Ellen (3 November 2017). "Astronauts who take long trips to space return with brains that have floated to the top of their skulls". Quartz. Retrieved 3 November 2017.
- ^ "Beckman physiological and cardiovascular monitoring system". Science History Institute. Retrieved 31 July 2019.
- ^ a b "When Space Makes You Dizzy". NASA. 2002. Archived from the original on 2009-08-26. Retrieved 2012-04-25.
- ^ a b Kerr, Richard (31 May 2013). "Radiation Will Make Astronauts' Trip to Mars Even Riskier". Science. 340 (6136): 1031. Bibcode:2013Sci...340.1031K. doi:10.1126/science.340.6136.1031. PMID 23723213.
- ^ a b Zeitlin, C.; et al. (31 May 2013). "Measurements of Energetic Particle Radiation in Transit to Mars on the Mars Science Laboratory". Science. 340 (6136): 1080–1084. Bibcode:2013Sci...340.1080Z. doi:10.1126/science.1235989. PMID 23723233. S2CID 604569.
- ^ a b Chang, Kenneth (30 May 2013). "Data Point to Radiation Risk for Travelers to Mars". New York Times. Retrieved 31 May 2013.
- ^ a b Gelling, Cristy (June 29, 2013). "Mars trip would deliver big radiation dose; Curiosity instrument confirms expectation of major exposures". Science News. 183 (13): 8. doi:10.1002/scin.5591831304. Retrieved July 8, 2013.
- ^ "Soviet cosmonauts burnt their eyes in space for USSR's glory". Pravda.Ru. 17 Dec 2008. Retrieved 2012-04-25.
- ^ a b c Dijk, Derk-Jan; Neri, David F.; Wyatt, James K.; Ronda, Joseph M.; Riel, Eymard; Ritz-De Cecco, Angela; Hughes, Rod J.; Elliott, Ann R.; Prisk, G. Kim; West, John B.; Czeisler, Charles A. (2001-11-01). "Sleep, performance, circadian rhythms, and light-dark cycles during two space shuttle flights". American Journal of Physiology. Regulatory, Integrative and Comparative Physiology. 281 (5): R1647–R1664. doi:10.1152/ajpregu.2001.281.5.R1647. ISSN 0363-6119. PMID 11641138. S2CID 3118573.
- ^ "Space Nursing Society". Retrieved 5 December 2011.
- ^ Perrin, MM (Sep 1985). "Space nursing. A professional challenge". Nurs Clin North Am. 20 (3): 497–503. doi:10.1016/S0029-6465(22)01894-1. PMID 3851391. S2CID 252060683.
- ^ "Ten-year astronaut sleep study reveals widespread use of sleeping pills in space". PBS NewsHour. 2014-08-07. Retrieved 2023-07-17.
- ^ Barger, Laura K; Flynn-Evans, Erin E; Kubey, Alan; Walsh, Lorcan; Ronda, Joseph M; Wang, Wei; Wright, Kenneth P; Czeisler, Charles A (September 2014). "Prevalence of sleep deficiency and use of hypnotic drugs in astronauts before, during, and after spaceflight: an observational study". The Lancet Neurology. 13 (9): 904–912. doi:10.1016/S1474-4422(14)70122-X. PMC 4188436. PMID 25127232.
- ^ Berry, C. A. (1967-07-24). "Space medicine in perspective. A critical review of the manned space program". JAMA. 201 (4): 232–241. doi:10.1001/jama.1967.03130040028009. ISSN 0098-7484. PMID 4383984.
- ^ "Blues Cues | Harvard Medicine Magazine". magazine.hms.harvard.edu. Retrieved 2023-07-17.
- ^ Paul, Ketema N.; Saafir, Talib B.; Tosini, Gianluca (December 2009). "The role of retinal photoreceptors in the regulation of circadian rhythms". Reviews in Endocrine and Metabolic Disorders. 10 (4): 271–278. doi:10.1007/s11154-009-9120-x. ISSN 1389-9155. PMC 2848671. PMID 19777353.
- ^ Enezi, Jazi al; Revell, Victoria; Brown, Timothy; Wynne, Jonathan; Schlangen, Luc; Lucas, Robert (August 2011). "A "Melanopic" Spectral Efficiency Function Predicts the Sensitivity of Melanopsin Photoreceptors to Polychromatic Lights". Journal of Biological Rhythms. 26 (4): 314–323. doi:10.1177/0748730411409719. ISSN 0748-7304. PMID 21775290. S2CID 22369861.
- ^ ScienceCasts: The Power of Light, retrieved 2023-07-17
- ^ Harbaugh, Jennifer (2016-10-19). "Let There Be (Better) Light". NASA. Retrieved 2023-07-17.
- ^ "Circadian Light for ISS".
- ^ designboom, matthew burgos | (2023-03-17). "SAGA's multicolored circadian light panel helps astronauts in space beat insomnia". designboom | architecture & design magazine. Retrieved 2023-07-17.
- ^ Dijk, D. J.; Neri, D. F.; Wyatt, J. K.; Ronda, J. M.; Riel, E.; Ritz-De Cecco, A.; Hughes, R. J.; Elliott, A. R.; Prisk, G. K.; West, J. B.; Czeisler, C. A. (November 2001). "Sleep, performance, circadian rhythms, and light-dark cycles during two space shuttle flights". American Journal of Physiology. Regulatory, Integrative and Comparative Physiology. 281 (5): R1647–1664. doi:10.1152/ajpregu.2001.281.5.R1647. ISSN 0363-6119. PMID 11641138. S2CID 3118573.
- ^ Gunja, Naren (June 2013). "In the Zzz Zone: The Effects of Z-Drugs on Human Performance and Driving". Journal of Medical Toxicology. 9 (2): 163–171. doi:10.1007/s13181-013-0294-y. ISSN 1556-9039. PMC 3657033. PMID 23456542.
- ^ "Effects of zolpidem and zaleplon on cognitive performance after emergent morning awakenings at Tmax: a randomized placebo-controlled trial". Retrieved 2023-07-17.
- ^ a b Guo, Jin-Hu; Qu, Wei-Min; Chen, Shan-Guang; Chen, Xiao-Ping; Lv, Ke; Huang, Zhi-Li; Wu, Yi-Lan (2014-10-21). "Keeping the right time in space: importance of circadian clock and sleep for physiology and performance of astronauts". Military Medical Research. 1 (1): 23. doi:10.1186/2054-9369-1-23. ISSN 2054-9369. PMC 4440601. PMID 26000169.
- ^ Ramburrun, Poornima; Ramburrun, Shivani; Choonara, Yahya E. (2022), Pathak, Yashwant V.; Araújo dos Santos, Marlise; Zea, Luis (eds.), "Sleep in Space Environment", Handbook of Space Pharmaceuticals, Cham: Springer International Publishing, pp. 469–483, Bibcode:2022hsp..book..469R, doi:10.1007/978-3-030-05526-4_33, ISBN 978-3-030-05526-4, retrieved 2023-07-17
- ^ Thirsk, R.; Kuipers, A.; Mukai, C.; Williams, D. (2009-06-09). "The space-flight environment: the International Space Station and beyond". Canadian Medical Association Journal. 180 (12): 1216–1220. doi:10.1503/cmaj.081125. ISSN 0820-3946. PMC 2691437. PMID 19487390.
- ^ Emanuelli, Matteo (2014-03-17). "Evolution of NASA Medical Kits: From Mercury to ISS". Space Safety Magazine. Retrieved 28 April 2015.
- ^ Gray, Tara. "John H. Glenn Jr". NASA History Program Office. Archived from the original on January 28, 2016. Retrieved December 9, 2016.
- ^ Wooley, Bennie (1972). "Apollo Experience Report- Protection of Life and Health" (PDF). NASA Technical Note: 20.
- ^ "Doctors remove tumour in first zero-g surgery".
- ^ "rHEALTH ONE flight demonstration".
- ^ Gahbauer, R., Koh, K. Y., Rodriguez-Antunez, A., Jelden, G. L., Turco, R. F., Horton, J., ... & Roberts, W. (1980). Preliminary results of fast neutron treatments in carcinoma of the pancreas.
- ^ Goldin D. S. (1995). "Keynote address: Second NASA/Uniformed Services University of Health Science International Conference on Telemedicine, Bethesda, Maryland". Journal of Medical Systems. 19 (1): 9–14. doi:10.1007/bf02257185. PMID 7790810. S2CID 11951292.
- ^ Maffiuletti, Nicola A.; Green, David A.; Vaz, Marco Aurelio; Dirks, Marlou L. (2019-08-13). "Neuromuscular Electrical Stimulation as a Potential Countermeasure for Skeletal Muscle Atrophy and Weakness During Human Spaceflight". Frontiers in Physiology. 10: 1031. doi:10.3389/fphys.2019.01031. ISSN 1664-042X. PMC 6700209. PMID 31456697.
- ^ Lake, David A. (1992-05-01). "Neuromuscular Electrical Stimulation". Sports Medicine. 13 (5): 320–336. doi:10.2165/00007256-199213050-00003. ISSN 1179-2035. PMID 1565927. S2CID 9708216.
- ^ a b c d "Critical Care Dialysis System" (PDF). NASA. Retrieved November 29, 2022.
- ^ "NASA - NASA's Lecture Series - Prof. Stephen Hawking".
- ^ a b c d e f g h i j k l m "Final Report: Research and Developmen of a Versatile Portable Speech Prosthesis" (PDF). Retrieved November 29, 2022.
- ^ a b c d e f Meltzner, G. S.; Heaton, J. T.; Deng, Y.; De Luca, G.; Roy, S. H.; Kline, J. C. (2018). "Development of sEMG sensors and algorithms for silent speech recognition". Journal of Neural Engineering. 15 (4): 046031. Bibcode:2018JNEng..15d6031M. doi:10.1088/1741-2552/aac965. PMC 6168082. PMID 29855428.
- ^ "Programmable Pacemaker". NASA. 1996. Retrieved September 25, 2022.
...the NASA-developed technology for two-way communication with satellites that provided a way for physicians to communicate with an implanted pacemaker and reprogram it without surgery.
- ^ "Hawking takes zero-gravity flight". BBC. 2007-04-27. Retrieved 2018-02-03.
- ^ "'Anti-Gravity' Treadmills Speed Rehabilitation". NASA. 2009. Retrieved September 25, 2022.
- ^ "Advanced Diagnostic Ultrasound in Microgravity (ADUM)". Nasa.gov. 2011-11-08. Archived from the original on 2007-08-23. Retrieved 2012-02-10.
- ^ Sishir Rao, BA (May 1, 2008). "A Pilot Study of Comprehensive Ultrasound Education at the Wayne State University School of Medicine". Journal of Ultrasound in Medicine. 27 (5). Lodewijk van Holsbeeck, BA, Joseph L. Musial, PhD, Alton Parker, MD, J. Antonio Bouffard, MD, Patrick Bridge, PhD, Matt Jackson, PhD and Scott A. Dulchavsky, MD, PhD: 745–749. doi:10.7863/jum.2008.27.5.745. PMID 18424650.
- ^ E. Michael Fincke, MS (February 2005). "Evaluation of Shoulder Integrity in Space: First Report of Musculoskeletal US on the International Space Station". Radiology. 234 (2). Gennady Padalka, MS, Doohi Lee, MD, Marnix van Holsbeeck, MD, Ashot E. Sargsyan, MD, Douglas R. Hamilton, MD, PhD, David Martin, RDMS, Shannon L. Melton, BS, Kellie McFarlin, MD and Scott A. Dulchavsky, MD, PhD: 319–322. doi:10.1148/radiol.2342041680. PMID 15533948.
- Sources
- MacPherson G (2007). "Altitude Decompression Sickness Susceptibility". Aviation, Space, and Environmental Medicine. 78 (6): 630–631. PMID 17571668.
- John-Baptiste A; Cook T; Straus S; Naglie G; et al. (2006). ""Decision Analysis in Aerospace Medicine " Costs and Benefits of a Hyperbaric Facility in Space". Aviation, Space, and Environmental Medicine. 77 (4): 434–443. PMID 16676656.
- DeGroot D; Devine JA; Fulco CS (2003). "Incidence of Adverse Reactions from 23,000 Exposures to Simulated Terrestrial Altitudes up to 8900 m". Aviation, Space, and Environmental Medicine. 74 (9): 994–997. PMID 14503681.
External links edit
- Space Medicine Association
- Description of space medicine Archived 2007-03-17 at the Wayback Machine
- NASA History Series Publications (many of which are online)
- Sleep in Space, Digital Sleep Recorder used by NASA in STS-90 and STS-95 missions
- A Solution for Medical Needs and Cramped Quarters in Space – NASA Archived 2016-04-12 at the Wayback Machine